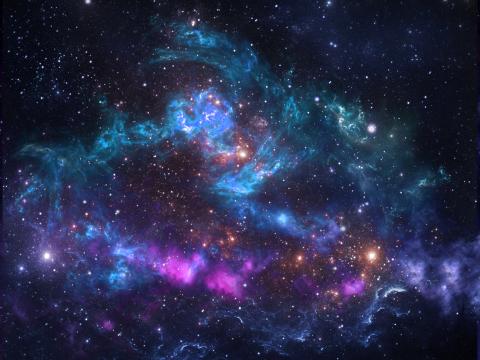
Dark energy, the mystery challenging cosmology
This article was originally published in L'Édition n°26.
For some six billion years, the expansion of the universe has been accelerated by a mysterious component known as "dark energy". But what exactly is dark energy? New experiments designed to scan the cosmos and involving researchers from Université Paris-Saclay, will attempt to determine this over the coming years.
The universe formed around 13.8 billion years ago, from a very dense and hot point that suddenly began to expand. At least, that's what the Big Bang theory describes. In the following moments, this primordial universe began to cool, creating favourable conditions for the appearance of the basic constituents of matter, quarks and electrons. These quickly grouped together to form the nuclei of future atoms and, a few hundred thousand years later, the first atoms. Gradually, this matter expanded and organised, giving rise to the first stars and galaxies and all the structures we know today. Since it formed, the universe has continued to evolve, but how has this happened? And what are the mechanisms driving this vast structure of the universe? These are some of the questions cosmology is trying to answer in a bid to piece together this story. This is a puzzle with many unknowns, some of which have only recently came to light and dark energy is one such unexplored phenomenon.
Scientists now estimate that the universe is comprised of 5% ordinary matter, 25% dark matter and 70% dark energy. However, a century earlier, neither of these last two components was part of the equation. The idea of dark matter emerged in the 1930s, when Swiss astronomer Fritz Zwicky noticed anomalies in the rotational speeds of objects in a cluster of galaxies. These speeds did not correspond to the estimated mass of the galaxy cluster. The discovery went unnoticed and was forgotten, only to resurface forty years later when American astronomer Vera Rubin made a similar observation on the scale of galaxies. As observations continued, the evidence mounted; the universe seemed to have far more matter than could be detected. This was when dark matter, a hypothetical form of matter that does not, or only barely, interacts with light, made its appearance, but what about dark energy? "The concept emerged in 1998 with the discovery of the accelerating expansion of the universe," explains Étienne Burtin, a researcher at the Division of Particle Physics (DPhP - Univ. Paris-Saclay/French Alternative Energies and Atomic Energy Commission, CEA).
Acceleration revealed by supernovæ
Until then, the universe was thought to be composed entirely of ordinary matter and dark matter. However, this component manifested itself in a gravitational effect that draws objects - stars, galaxies, etc. - towards each other. "The more matter there is, the stronger the gravity holding objects together. The expansion of the universe was therefore expected to slow gradually and for there to be a direct relationship between this deceleration and the mass holding the structures together," confirms Jérémy Neveu, a lecturer at the Laboratory of the Physics of the two Infinities - Irène Joliot-Curie (IJCLab - Univ. Paris-Saclay/Univ. Paris-Cité/National Centre for Scientific Research, CNRS).
In the late 1990s, a team of scientists set out to measure the slowing of the expansion and thus estimate the density of matter in the universe by observing type Ia supernovae. These explosions of stars at the end of their lives are unique because they emit a relatively consistent and observed luminous flux. Since the amount of light received by an observer is related to its distance from the emitting star, it is possible to estimate the distance of supernovae from the observed light intensity. And there was a surprise in store; the supernovae studied appeared to be much further away than expected. Shortly afterwards, a second group of researchers obtained similar results with other supernovae. The scientific community realised that the universe was still expanding and this expansion was accelerating. This discovery - which earned the Americans Saul Permutter, Adam Riess and Brian P. Schmidt the Nobel Prize in Physics in 2011 - "wasn't totally unexpected," says Étienne Burtin. "Some research had shown that a universe composed solely of matter did not align well with the observations."
How can this acceleration be explained? This is where dark energy comes into play. This component would act in opposition to gravitation, pushing objects away from each other. According to estimates, this "late-time" acceleration began around 6.5 billion years after the Big Bang. "It's a rather strange effect due to the expansion of the universe," explains Jérémy Neveu. "As the Universe expands, matter becomes diluted and less effective at slowing the expansion. Since dark energy is always present, it eventually takes over and accelerates the expansion." Described like this, the process seems straightforward. However, there is much debate as to the exact nature of this component. "When we talk about dark energy, we're overestimating because we don't know if it's energy," stresses Étienne Burtin. In reality, "we simply wanted a word to describe a phenomenon we don't understand and which remains open to discussion."
Cosmological constant, quintessence... Various theories
Since this acceleration was discovered, various theories on dark energy have emerged, some more convincing than others. The first candidate is the cosmological constant (or lambda constant, Λ). Conceived by Albert Einstein in 1917, it was incorporated into the equations of general relativity, which describe how matter and energy distort space-time. The physicist introduced it "because he thought the universe was static," explains Étienne Burtin. "To obtain something static without changing his equations, he added a cosmological constant." But after studies confirmed a dynamic, expanding universe, Einstein eventually abandoned his constant. Nearly a century later, it is back in the spotlight with the mystery of dark energy and the Lambda-CDM model. This assumes that the universe includes both matter, known as Cold Dark Matter (CDM), and dark energy in the form of a fundamental constant (Lambda).
"This is the model with the strongest explanatory power because it's so simple. It's simply a case of adding a constant to the equations to almost explain the acceleration measured today," says the DPhP researcher. Almost, because problems remain, not least the value of this constant. According to this theory, "dark energy arises from vacuum energy. The universe is expanding, which creates volume, and each added volume of space comes with vacuum energy." According to quantum physics, the vacuum is not empty but full of particles that emerge and disappear just as quickly. "The problem is that estimating this energy using the standard model of particle physics leads to results 1055 to 10120 larger than what we measure from the observations!" A considerable flaw for the cosmological constant.
Another possibility suggests that dark energy is a dynamic entity, i.e. a component that varies as a function of time and space. A model known as "quintessence" proposes that the mysterious energy is the manifestation of a new form of fundamental force induced by unknown particles. "There are also so-called modified gravity theories, which assume that gravitation as described by the general relativity model would not work on the cosmological scale," adds the physicist. "Consequently, dark energy would not be a new substance to be added to the universe, but rather an improvement to be made to the known gravity model."
5,000 robotic eyes searching for dark energy
The question of dark energy has now become a real investigation for cosmologists. While several experiments have already provided clues, a new era has recently begun with the launch of three large-scale missions. The first is the DESI (Dark Energy Spectroscopic Instrument) project conducted on the Mayall telescope at Kitt Peak Observatory in Arizona (USA). Commissioned in 2021, this instrument is equipped with a four-meter mirror where the focal plane is lined with 5,000 robotic fibre optic positioners connected to ten spectrographs. "In a single telescope sighting, DESI records the spectra of 5,000 astrophysical objects," says Étienne Burtin, a member of the international group that is working on the instrument and brings together over seventy institutions. By way of comparison, the SDSS (Sloan Digital Sky Survey), which preceded DESI, "only" captured a thousand objects at each observation. Importantly, this worked with shutter plates that had to be changed manually. DESI offers "enormous savings in terms of time and infrastructure," stresses the researcher.
Its aim is to observe tens of millions of galaxies and quasars - very luminous objects - in order to build a detailed three-dimensional map of the universe. With this survey, "the aim is to trace the history of the expansion of the universe and measure its speed at different periods over the last eleven billion years." To make these measurements, scientists use a special feature called Baryonic Acoustic Oscillations (BAO), the origins of which date back to the primordial universe. At that time, the universe was a hot, dense plasma filled with interacting particles, notably baryons (protons and neutrons), within which small fluctuations appeared. These generated pressure waves, overdensities of matter, which propagated step by step until freezing as the universe cooled and the first atoms formed. Billions of years later, this phenomenon has left its mark on the spatial distribution of galaxies, providing scientists with a "cosmic rule". "Since they were frozen, these overdensities have only been subject to the expansion of the universe. They provide us with a characteristic distance scale for measuring the speed at which the universe has expanded so far," says Étienne Burtin.
After just one year, the DESI spectrographs have revealed the distance to over six million galaxies and quasars where the light has taken between one and eleven billion years to reach Earth. This first sample is proving invaluable in testing the various theories being considered. In April 2024, an initial study indicated that the observations from DESI seemed to support the Lambda-CDM model and thus the existence of a universe including dark matter and energy. However, it also highlights deviations suggesting variations in dark energy over the history of the universe. "These observations are interesting because if dark energy varies over time, this rules out the possibility of a cosmological constant. But we need to analyse more data for more robust results," explains Étienne Burtin. In November 2024, a second study confirmed that gravity behaves in accordance with the model of general relativity on cosmological scales, which would tend to rule out the possibility of modified gravity.
The DESI mission, which is set to continue until 2026, is the first fully blind spectroscopic experiment. For each study, scientists work with modified data to avoid biases that could influence their interpretation. "The positions of the galaxies and quasars we analyse are consistently modified to hide the underlying cosmological parameters," confirms the physicist. "This is very important for an honest analysis. It also gives us more confidence in the results." The team is currently studying the first three years of DESI data, and is due to publish its analysis in March 2025.
A space telescope as a cosmic detective
DESI may be leading the way, but the instrument is not alone in this dark quest. "What's interesting in our field is the combination of experiments. Each one tells us something, but it's only when you combine all the results that you really obtain information," highlights Étienne Burtin. Since July 2023, another unique experiment has been set up in space, some 1.5 million kilometres from Earth: the Euclid space telescope. Operated by the European Space Agency (ESA), the spacecraft's mission is to observe 1.5 billion galaxies located up to ten billion light-years away (9.46 x 1012 kilometres), covering one-third of the celestial sphere. "That's about 15,000 square degrees," explains Martin Kilbinger, a researcher at the Department of Astrophysics (DAp - Univ. Paris-Saclay/CEA) and a member of the international consortium of over 2,500 scientists working on the telescope.
Euclid has two onboard instruments, a Visible Instrument (VIS) and a Near-Infrared Spectrometer and Photometer (NISP), to observe galaxies. Using the VIS, cosmologists are studying the so-called gravitational lensing effect. "Light from galaxies doesn't travel in a totally straight line," the researcher explains. "Its direction changes due to matter in its path, which distorts space-time. This creates small distortions in the images of galaxies. This is known as gravitational shear." By subjecting the observations to algorithms, it is not only possible to measure these distortions, but also to determine the distribution of the matter that causes them. Similarly, these measurements open a window on the evolution of this matter as a function of time and on the potential effects of dark energy. In this mission, Euclid has a major advantage over other instruments; it is located in space, so its observations are not affected by the Earth's highly turbulent atmosphere, which has its own effects on images of galaxies.
The second instrument, the NISP, focuses on another phenomenon known as redshift. As galaxies move further apart, the light waves they emit shift towards longer wavelengths in the spectrum, i.e. towards the red. By analysing this shift, it is possible to measure the distance to distant galaxies, as well as their motion. Due to the combination of the data from the VIS and NISP, which will be analysed blind, scientists plan to draw up their own 3D map of the universe at different periods in order to trace its history. One of Euclid's strengths lies in "the enormous amount of data it gives us on galaxies," stresses Martin Kilbinger. "We want to compare the observations with the models and their theoretical predictions of the expansion of the universe, the rate of matter, the Λ constant, and so on."
It's still too early to say which dark energy theory will stand up using Euclid. Although the telescope delivered its first spectacular images at the end of 2023, its main survey did not start until summer 2024, due to technical problems that delayed operations. "We've started analysing the first six months of data," says the cosmologist. "We'll publish the main results in 2026 and I'm pretty confident that we'll produce constraints on the Λ constant, just as DESI did." Although DESI seems to discredit the cosmological constant, this is the constant that most convinces the researcher: "I'm quite pessimistic, I don't think dark energy is going to be a real discovery." Proof that the question of the existence of this mysterious energy still sparks debate among scientists, who are eagerly awaiting the next measurements.
The largest astronomical camera ever built
For Jérémy Neveu, at the IJCLab, "dark energy is the most important question in cosmology today." Furthermore, he makes no secret of his enthusiasm at seeing certain results contradict the simplest path of the cosmological constant. But the investigation is still in its early stages. To solve the mystery, the lecturer is also participating in a global project: the Vera C. Rubin Observatory, which is set to deliver its first images in spring 2025. Sitting at an altitude of approximately 2,700 m on a mountain in Chile, this observatory houses three mirrors, giving it a very wide field of observation: an area equivalent to forty times that of the full Moon. However, that is not the only special feature; it is also equipped with the largest astronomical camera ever constructed - a device the size of a small car - with a resolution of 3.2 billion pixels and six colour filters.
"With the Vera C. Rubin Observatory, we've tried to build the most complete ground-based telescope for modern cosmology," says Jérémy Neveu. "The aim is to look at the universe from every angle. We're going to hunt down the presence of dark energy and dark matter by observing type Ia supernovae, looking at the distribution of matter and studying the gravitational lensing effect in particular. This telescope is one of the first built to make this measurement." Using its instruments, the Legacy Survey of Space and Time (LSST) experiment plans to survey the entire visible southern sky every three to four nights, at a rate of 800 images per night.
"To give an order of magnitude, the 1998 discovery was made with around forty Ia supernovae. Today, around 2,000 are catalogued. LSST plans to gather 100,000 of them over a ten-year mission. And there won't just be more of these supernovae, they'll also be better measured," emphasises Jérémy Neveu. "The advantage of this observatory is that it can scan the entire sky in three days and since a supernova lasts an average of one to two months, this provides a great chance to observe many of them and increase the number of observations of the same supernova." In ten years, some seventeen billion stars and twenty billion galaxies will be added to the inventory observed by LSST, shedding light on the dark side of the universe, but not just that.
With the Chilean observatory, as with DESI or Euclid, scientists also hope to make progress on other cosmological questions that currently remain unsolved, such as the mass of neutrinos or the tension on the Hubble constant, which is the discrepancy between different methods of measuring the speed of expansion of the universe. "The question of dark energy is interesting because it paves the way to other mysteries in the history of the primordial universe, such as inflation," adds Étienne Burtin, who is already considering missions post-DESI. Whether or not these ground-breaking missions result in a genuine discovery of dark energy, "cosmology undoubtedly has a bright future ahead," concludes Jérémy Neveu.
References :
- DESI Collaboration et al., DESI 2024 VII: Cosmological Constraints from the Full-Shape Modeling of Clustering Measurements, arXiv, 2024.
- Scaramella et al., Euclid preparation: I. the Euclid Wide Survey, Astronomy and Astrophysics, 2022.
- Neveu et al., On the importance of Earth's atmosphere for SNIa precision cosmology, arXiv, 2024.
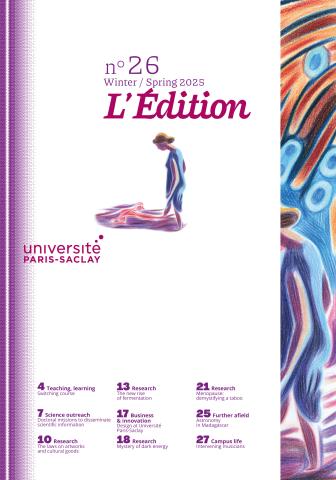
This article was originally published in L'Édition n°26.
Find out more about the journal in digital version here.
For more articles and topics, subscribe to L'Édition and receive future issues: